In-depth Analysis! Seven Major Orthopedic Biomedical Materials
Orthopedic biomaterials is a more general term that encompasses all biocompatible materials used in orthopedic surgery and treatment. In addition to the functions of bone repair materials, these materials may also be used to support, replace, or repair other tissues such as tendons, cartilage, and muscles. From fracture repair to joint reconstruction, from cartilage repair to tendon regeneration, each step of the treatment process may involve one or more specific biomaterials.
This article will provide a detailed introduction to seven major types of orthopedic biomedical materials, which include:
-
Bone substitute materials: These materials are used to replace or repair damaged bones and are designed to mimic the structure and function of natural bone. -
Bone graft materials: encompassing various materials from autologous and allogeneic to xenogeneic bone grafts, used to fill bone defects or enhance bone healing. -
Cartilage substitutes and graft materials: designed specifically for repairing or replacing damaged cartilage, these materials help restore joint function and reduce pain. -
Tendon tissue substitutes and graft materials: Used to repair or replace injured tendons, these materials need to possess high elasticity and strength to support dynamic loads. -
Orthopedic internal fixation materials: including various nails, rods, plates, and other devices used for the internal fixation of fractures or in bone reconstruction surgeries. -
Orthopedic external fixator brackets and applications: These devices stabilize fractures or correct deformities through external brackets, and the design of the brackets should accommodate the specific needs of the patient. -
Orthopedic bioabsorbable internal fixation materials: These materials gradually degrade and absorb within the body, eliminating the need for a second surgery to remove them, while providing sufficient initial strength to support bone healing.
Bone substitute materials
-
Bone Screws: Used to fix fracture fragments or as lag screws to hold fracture pieces together, common types include PDLLA/HA composite absorbable bone screws and metal titanium alloy bone screws.
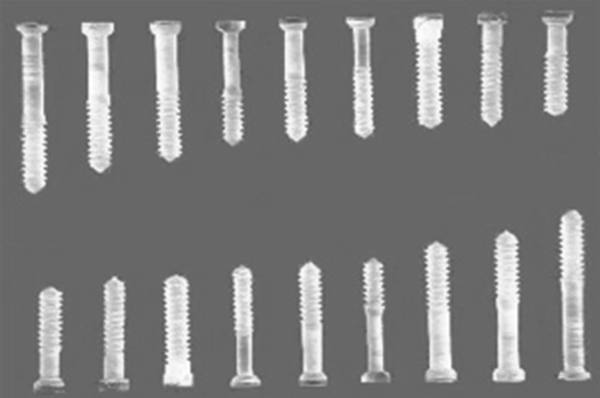
-
Bone plates: closely attached to the bone to provide fixation, used in conjunction with screws for internal fixation of fractures, such as straight and irregular metal bone plates, as well as special-shaped bone plates like herringbone, arc-shaped, L-shaped, etc.
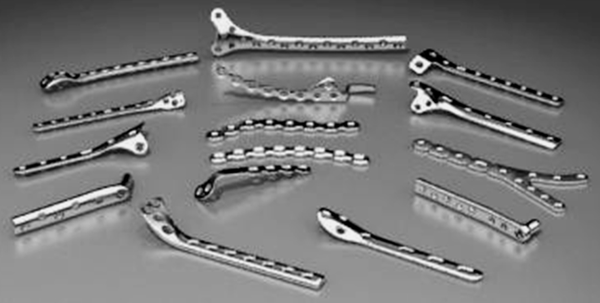
-
Intramedullary nails: used for internal fixation of fractures, especially long bone fractures, such as V-shaped intramedullary nails, cloverleaf intramedullary nails, and interlocking intramedullary nails.
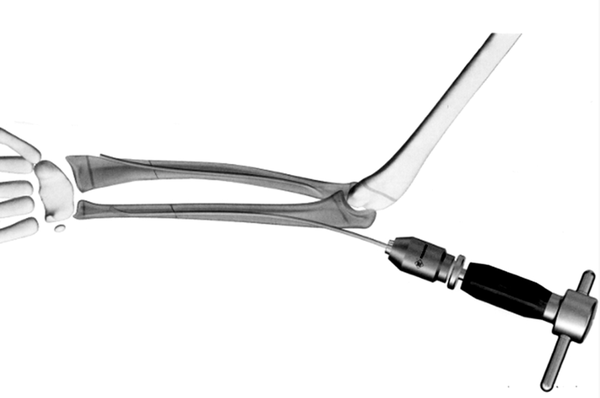
-
Spinal implants: used for spinal stabilization and fusion, including interbody fusion devices, spinal fixation systems (such as pedicle screw systems), artificial intervertebral discs, etc.
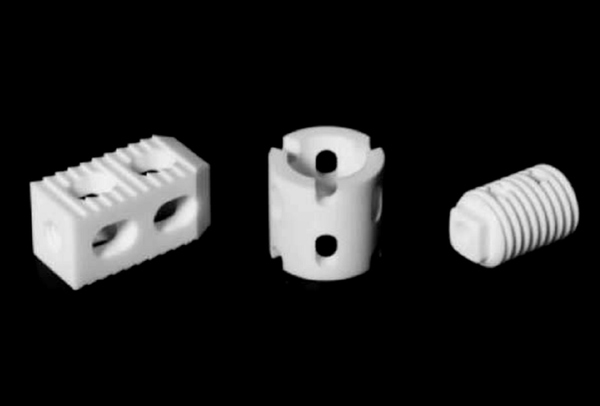
-
Artificial joints: hip joints, knee joints, shoulder joints, elbow joints, ankle joints, wrist joints, finger joints, etc. Among them, artificial hip joints and artificial knee joints are the most developed and widely used.
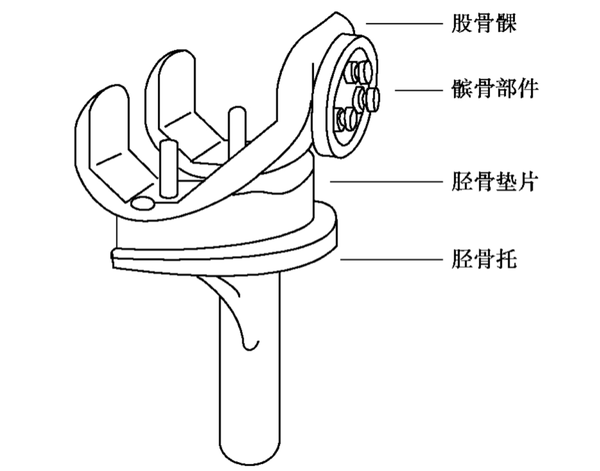
These orthopedic implants have a wide range of applications in clinical practice. They help restore the structure and function of bones by providing stable fixation and support. With advancements in biomaterials and manufacturing technologies, the design and materials of these implants are continuously optimized to improve their biocompatibility, mechanical properties, and ability to promote bone healing.
The main characteristics of bone substitute materials include:-
Biocompatibility: The material should not cause adverse reactions or immune rejection in the host body. -
Bioactivity: Some bone substitute materials possess the ability to promote the proliferation and differentiation of bone cells, thereby accelerating the regeneration of bone tissue. -
Plasticity: The material should be easy to shape or customize to adapt to the specific anatomical structures and surgical needs of different patients. -
Mechanical properties: The material should have sufficient strength and toughness to support bone tissue growth, while its elastic modulus should be close to that of human bone to prevent stress shielding effects. -
Biodegradability: Many bone substitute materials are designed to be naturally degraded and absorbed in the body, so that they can be replaced by newly formed bone tissue over time.
Bone graft materials
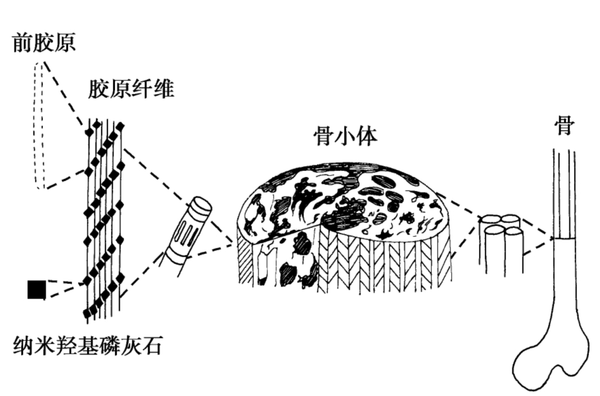
Bone graft materials are biomedically engineered materials used to repair or replace damaged bone tissue. These materials are designed to fill bone defects or provide structural support for bone tissue, promoting bone healing and regeneration. Bone graft materials can be autologous (from the patient's own bone tissue), allogeneic (from the bone tissue of other individuals of the same species), xenogeneic (from other species, such as cows or pigs), or synthetic (artificially manufactured materials).
Natural bone grafts:Natural bone grafts are commonly used materials in orthopedic and dental surgeries for repairing or reconstructing bone defects and injuries. These materials are derived from natural sources, providing excellent biocompatibility and bioactivity. The following is a detailed introduction to natural bone grafts, including the characteristics and applications of autografts, allografts, xenografts, and naturally derived bone materials.
Autologous bone, allogeneic bone, and xenogeneic bone
Autograft
Autologous bone grafting refers to the transplantation of bone from one part of a patient's body to another. This type of graft is considered the ideal bone grafting material due to its optimal biocompatibility and osteogenic capacity, as it does not trigger an immune response. Autologous bone grafting can be further divided into non-vascularized autologous bone grafting and vascularized autologous bone grafting. Non-vascularized grafting is relatively simple to perform and thus was adopted early in clinical practice. With advancements in microsurgical techniques, vascularized grafting was developed, which involves vascular anastomosis to maintain adequate blood supply to the graft, preserving its regenerative potential. This significantly improves the success rate of the graft and enhances the quality of autologous bone transplantation. Common sources for non-vascularized autologous bone grafts include the ilium, tibia, and calvaria. For vascularized autologous bone grafts, commonly used sources include the scapular osteocutaneous flap, fibular osteocutaneous flap, latissimus dorsi osteocutaneous flap, and iliac osteocutaneous flap.
-
Advantages: No immune rejection reactions, high osteogenic capability. -
Disadvantages: The bone graft site may develop complications, such as pain and infection; the available bone quantity is limited.
Allograft
Allogeneic bone is derived from another individual of the same species, typically from a donor. This type of bone graft performs well in terms of osteoconductivity and can retain a certain degree of osteoinductivity after processing. Sources for allogeneic bone include: (1) bone tissue from amputated limbs; (2) ribs removed during thoracic surgery; (3) bones from fresh cadavers, with cartilage often used from deceased infants. Bone tissue from patients with tumors, infectious diseases, bacterial infections, skeletal disorders, or blood diseases is prohibited for collection. Depending on the type of graft, allogeneic bone transplantation can be classified as allogeneic bone grafting, allogeneic cartilage grafting, or allogeneic bone joint grafting. Based on different processing methods, there are fresh allogeneic bone, banked bone, and demineralized bone matrix gelatin. Allogeneic bone transplantation can avoid some of the drawbacks of autologous bone transplantation, but the main issues are the risks of rejection and cross-infection. Therefore, allogeneic bone needs to be processed before use, with the purpose of reducing or eliminating its immunogenicity. However, various processing methods may cause varying degrees of damage to, or even the death of, the bone cells in the allogeneic bone. The osteobiological effects of allogeneic bone in the host site mainly manifest as osteoconductivity and osteoinductivity. After processing, allogeneic bone becomes dead bone and gradually gets absorbed upon contacting the host bone bed. New bone growth occurs through this absorption process, where the allogeneic bone is "creeping substituted" by osteoblasts from the host bone and periosteum, resulting in the formation of new bone.
-
Advantages: can be used for large bone defects and provides good mechanical support. -
Disadvantages: may trigger immune reactions, risk of disease transmission; requires strict screening and processing. -
Processing methods include fresh bone, deep-frozen bone, and freeze-dried bone. Freeze-dried bone (lyophilized bone) is generally preferred due to its lower immunogenicity.
Xenograft bone refers to bone material derived from other species. These materials need to undergo special processing to reduce immune responses and the risk of disease transmission. The main sources of xenograft bone materials include bovine bone, porcine bone, deer bone, sheep bone, etc. Among these, porcine and bovine bone materials are more readily available and have been studied the most in xenograft bone research. Currently, a relatively consistent view on xenograft bone is that both immune reactivity and osteoinductive activity share a common material basis; eliminating antigenicity also destroys the substances that induce bone formation. Therefore, pure xenograft bone cannot resolve the contradiction between eliminating antigenicity and maintaining osteoinductive activity. Combining the bone-active substances of de-antigenized xenograft bone to produce composite xenograft bone can partially restore the osteoinductive capability of xenograft bone, thereby addressing some of the difficulties brought about by this issue. This approach has become a new direction in xenograft bone transplantation research. For example, xenograft bone combined with bone morphogenetic protein (BMP), xenograft bone combined with autologous red bone marrow, xenograft bone combined with bone matrix gelatin, as well as xenograft bone combined with various growth factors.
-
Advantages: Widely available, suitable for applications that do not require high mechanical strength. -
Disadvantages: The issues of immune response and biocompatibility are more prominent and require meticulous handling.
Bone-derived materials
Bone scaffold material
-
Advantages:
-
Disadvantages:
Lack of osteoinductivity: High-temperature treatment destroys the bioactive components in natural bone.
-
Advantages: Similar to the porous structure of human bone: Its structure mimics the cancellous structure of human bone, which is conducive to the growth of new bone. Suitable for bone ingrowth: The pore size is suitable for the inward growth of new bone. -
Drawback: Limited mechanical properties, although it has certain compressive strength, its tensile and shear strengths are relatively low.
-
Promoting bone healing: rich in growth factors and components that stimulate blood vessel growth. -
Wide application: It is commonly used as an adhesive, mixed with other bone substitutes to enhance the overall performance of the composite material. -
Application: Commonly used as an autologous bone graft extender or mixed with other materials such as hydroxyapatite.
-
Its advantages are as follows:
-
Its disadvantages are as follows:
These bone-derived materials each have unique characteristics and are widely used in bone repair and regeneration treatments in orthopedics and dentistry. The selection of suitable materials depends on specific clinical needs, anticipated biological functions, and the patient's particular circumstances. With advances in materials science, more efficient and biologically active novel bone-derived materials may be developed in the future.
Synthetic bone grafts play an increasingly important role in modern medicine, particularly in orthopedic and dental surgeries. The development of these materials aims to mimic the functions of natural bone while avoiding some of the limitations and risks associated with autologous and allogeneic bone grafts. Synthetic bone grafts can be roughly classified into inorganic bone graft materials, organic bone graft materials, and composite bone graft materials based on their composition and properties.
Dihydrate calcium sulfate, due to its fast curing speed, is often made into an injectable form. When using it, attention should be paid to ensure that the calcium sulfate adheres closely to the viable outer or inner periosteum of the bone so that it can serve as a bone-conductive matrix for vascular ingrowth. Calcium sulfate dissolves and is resorbed in the body within 5 weeks. Based on this characteristic, calcium sulfate can be used as a slow-release carrier for antibiotics in the treatment of osteomyelitis.
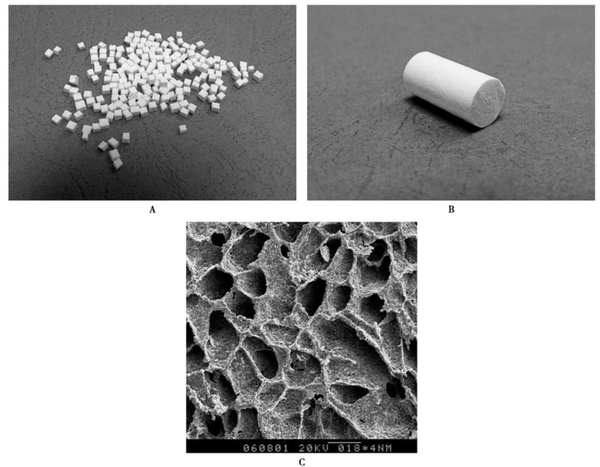
Fourthly, the strength of the composite material is close to that of cancellous bone and can be adjusted as needed, making it easy to shape with a surgical knife and highly convenient for clinical use. Clinical usage indicates that it has good biocompatibility with the human body, no immune rejection reactions, and favorable healing outcomes, making it a safe and effective new bone graft material.
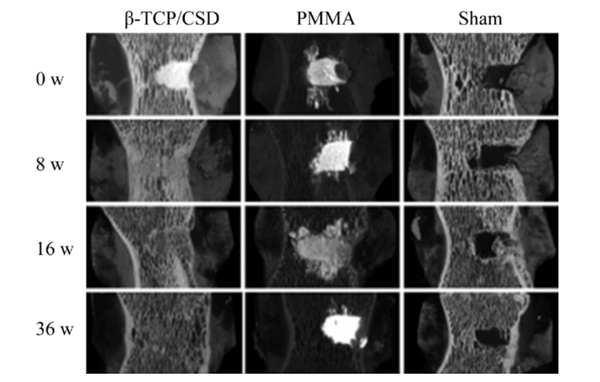
Calcium sulfate and inorganic ceramic materials primarily form stable structures through physical connections, with the mechanical strength of the composite being influenced by the type of materials, crystal phases, and proportions. The structure of calcium sulfate/hydroxyapatite is mainly maintained by the calcium sulfate matrix, with hydroxyapatite simply integrated into it. Therefore, as the calcium sulfate content decreases, the mechanical strength tends to decline. Among all the crystal phases of calcium sulfate, hemihydrate calcium sulfate can rapidly self-cure into the harder dihydrate calcium sulfate through hydration, which is of significant importance for early weight-bearing in clinical bone defect reconstruction.
Composite materials not only provide structural support and osteoconductivity, but also exhibit certain osteoinductive properties, and perform better in terms of immune rejection responses. The development and application of these materials have greatly enriched the options for bone repair, enhancing the flexibility and effectiveness of treatments. Through ongoing research and technological advancements, synthetic bone graft materials will play an increasingly crucial role in future medical applications.
Tissue-engineered bone
Cartilage replacement and transplantation materials
Chondrocytes, also known as chondrocytes, are typically round or oval-shaped and are located in small cavities called lacunae. These cells are responsible for forming fibers and secreting the matrix, playing an active role in cartilage growth and maintenance. The region surrounding the chondrocytes in the lacunae is rich in chondroitin sulfate and is called the chondron. This structure helps protect the cells and facilitates material exchange with the surrounding matrix.
Fibrous cartilage membrane: The exterior of the cartilage is wrapped in a layer of fibrous cartilage membrane, which is a type of tougher connective tissue that provides additional support and protection. It helps the cartilage bear loads and connect with adjacent bone structures.
Extracellular matrix: The extracellular matrix of cartilage is its main component, consisting of collagen, proteoglycans, hyaluronic acid, and liquid-phase components such as water and electrolytes. This complex network not only supports the chondrocytes but also provides the necessary microenvironment for cell growth. The high water content of the matrix and the properties of hyaluronic acid allow substances to freely permeate through the matrix, providing nutrients to deep chondrocytes even in avascular conditions.
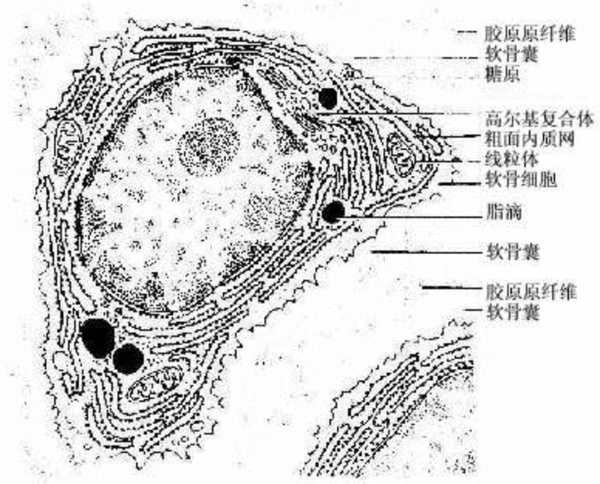
-
Transparent cartilage is the most common type of cartilage, and its main chemical components are proteoglycans, particularly long chains of hyaluronic acid, with many shorter proteoglycan side chains attached to them. These side chains are mainly chondroitin sulfate, which bind to type I collagen fibers, forming a mesh-like structure capable of withstanding pressure. Transparent cartilage does not have periodic cross-striations or form distinct bundles of collagen fibers, but its structure is sufficient to withstand considerable pressure and tension. -
Elastic cartilage contains a large amount of elastic fibers, giving it greater flexibility and elasticity. This type of cartilage is mainly found in the ears and laryngeal structures. -
Fibrocartilage contains a higher amount of collagen fibers, providing greater support and resistance to tension. It is primarily found in areas that endure heavy pressure, such as the meniscus in the knee and intervertebral discs.
In general, these components and properties of cartilage enable it to effectively withstand pressure and bending, while providing crucial structural support in joints and the skeletal system.
Autologous chondrocyte transplantation is a method that involves obtaining healthy chondrocytes from the patient's own non-weight-bearing areas, which are then cultured and expanded in vitro before being implanted into the damaged cartilage area. This method has been shown to maintain the integrity of the subchondral bone and to inhibit fibrosis caused by fibroblasts. Allogeneic chondrocyte transplantation shows similar repair effects to autologous chondrocyte transplantation, but immune response and cell preservation are its main issues.
Artificial cartilage replacement materials should possess good biomechanical properties, excellent lubricity and wear resistance, chondrocyte growth induction, strong bonding with the bone base, and biocompatibility. Currently used highly elastic materials such as silicone rubber, polyurethane, and polyvinyl alcohol hydrogel each have their own advantages and disadvantages. For instance, silicone rubber is prone to aging and failure, and the degradation performance of polyurethane needs improvement. The research focus is on improving existing materials and preparation processes, as well as exploring new materials.
-
Easy to obtain, abundant in source, and causes minimal damage to the organism. -
Strong proliferation ability in vitro culture. -
It has good adhesion to the bracket material. -
The seeded cells can adapt to the internal environment of the human body and maintain the characteristics of original chondrocytes.
Current research mainly focuses on autologous chondrocytes, allogeneic chondrocytes, mesenchymal stem cells, and embryonic stem cells. The selection of scaffold materials includes both naturally sourced biomaterials and artificially synthesized scaffold materials. The key lies in the design of the scaffold and the correct choice of materials to ensure the mechanical stability of the scaffold and promote the proliferation and migration of seeded cells.
Tendon Tissue Substitutes and Transplant Materials
-
Collagen bundles: These are the main components of tendons, consisting of a large number of collagen fibers arranged in parallel. This arrangement of fibers gives tendons a high resistance to stretching, allowing them to withstand the forces generated by muscles. -
Tendon gelatin: It is the matrix filled between collagen fibers, containing proteoglycans and water, helping the tendon maintain structural stability and elasticity when subjected to pressure. -
Tendon cells: mainly composed of fibroblasts and stromal cells, are distributed between collagen fibers, responsible for synthesizing and secreting collagen and other matrix components, maintaining the structure and function of tendons.
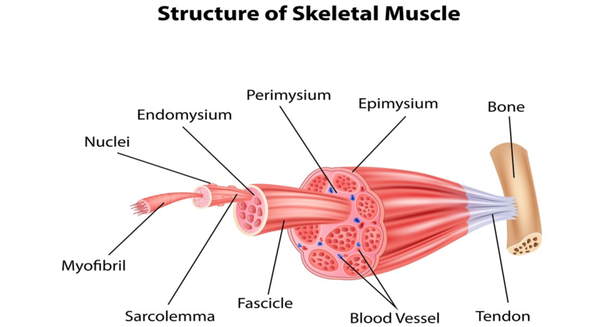
The self-repair ability of tendons is very limited, primarily because tendons have relatively poor blood supply, resulting in a slow healing process that often fails to fully restore their original structure and function. In clinical practice, severe tendon injuries may require surgical repair, including suturing torn tendons or using grafts to replace damaged portions.
Tendon injuries are common sports injuries, especially in cases of high-intensity or repetitive muscle use. Modern medicine has developed various methods for tendon injury repair and replacement, primarily including autologous tendon transplantation, allogeneic tendon transplantation, xenogeneic tendon transplantation, and artificial tendon substitutes.
Allograft tendon transplantation uses tendons from the same race but different individuals, expanding the pool of tendons available for transplantation. However, studies have shown that this method has a relatively high failure rate, with major issues including necrosis and rejection of the implanted tendon. Additionally, there is a risk of donor-transmitted viral infections, such as hepatitis and AIDS, which limits its clinical application.
Heterologous tendon transplantation utilizes tendons from different species. This method theoretically provides an abundant source of tendons, but immune rejection and biocompatibility issues remain significant challenges. Although chemical treatments such as the use of polyformaldehyde, freezing, and glutaraldehyde can reduce the immunogenicity of tendons, these processing methods may alter the biomechanical properties of the tendons, affecting the repair outcomes.
Artificial Tendon Substitute
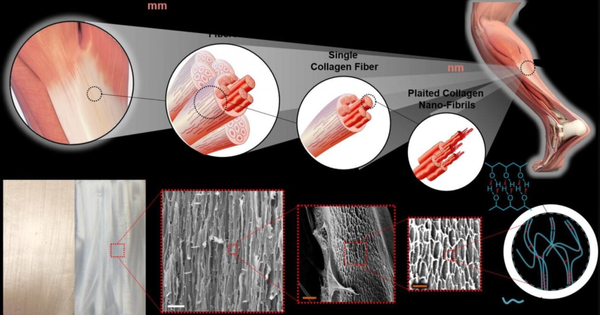
-
Good biological adaptability: Human hair keratin artificial tendons have good biocompatibility, able to gradually undergo tendon-like transformation in the body and integrate without significant adhesion to surrounding tissues. -
No immune rejection response: Due to special treatment, this artificial tendon does not trigger an immune rejection response in the body. -
Persistent mechanical properties: no attenuation of tensile stress, able to withstand long-term muscle movement traction. -
Tendonization Engineering: The process of being absorbed in the body while simultaneously forming new autologous tendons is referred to as tendonization engineering.
The development of this material marks an important advancement in artificial tendon technology, particularly in enhancing the functionality of tendon repairs and reducing surgical complications.
-
Naturalized repair at a high level: The formed tendon tissue is vibrant and functional, capable of achieving permanent replacement. -
Morphological and functional perfection reconstruction: It can be shaped according to the specific morphology of the defective tendon, achieving a highly matched morphological repair and functional reconstruction. -
No immune response or pathogen transmission risk: The seed cells used can be autologous cells, reducing the risk of immune rejection and disease transmission.
The key to tissue engineering technology lies in selecting appropriate seed cells and scaffold materials, as well as effectively combining the seed cells with the scaffold materials. The scaffold materials must not only possess good mechanical strength to support early activity but also degrade in sync with cellular function, providing space for cell growth and physiological function.
Orthopedic internal fixation materials
|
|
|
---|---|---|
|
|
|
|
|
|
|
|
|
|
|
|
-
Compressive force: Transmitted axially, increases bone loading, commonly seen in compressive fractures of the spine. -
Tension: Similarly transmitted through the axial direction, leading to fracture separation. -
Bending force: causes one side of the bone to bear pressure while the opposite side bears tension. -
Torsional force: A force that causes bones to withstand rotational stress. -
Shear force: caused by compressive force, leading to oblique fractures.
-
Stainless steel: It has good mechanical properties and cost-effectiveness, is easy to process, and is widely used in the manufacture of various internal fixation devices. It has relatively high bioactivity but may corrode in high-load environments. -
Cobalt-chromium-molybdenum alloy: low bioactivity, extremely high corrosion resistance and mechanical strength, but high cost and difficult processing. -
Titanium alloy: lightweight, highly corrosion-resistant, with excellent biocompatibility and low bioactivity. The elasticity of titanium alloy is similar to that of human bone, making it suitable for manufacturing long-term implants. It produces almost no artifacts in CT and MRI scans, facilitating follow-up examinations. -
Polylactic acid-based degradable materials: Good biocompatibility, can naturally degrade into water and carbon dioxide after use, eliminating the need for secondary surgery to remove them. Suitable for internal fixation scenarios that do not bear high loads. Their long-term biosafety is still under research.
The material should be biocompatible, not causing toxic reactions, inflammation, fibrosis, or macrophage activation. These biological responses may lead to pain, swelling, and dysfunction in the implantation area, and in rare cases, may even result in tumor formation. Therefore, for young individuals, it is generally recommended to remove the internal fixation device once the fracture has healed.
Implants should not rust or undergo electrolysis. When using stainless steel, it is important to ensure high purity and freedom from impurities to prevent corrosion. Additionally, measures should be taken to prevent contact between different internal fixation materials to avoid interfacial corrosion. Using internal fixation components made of the same material is also an effective method to prevent electrolytic corrosion.
Evolution of Internal Fixation Techniques
-
Anatomical reduction of the fracture ends: Especially for intra-articular fractures, it is emphasized that perfect anatomical reduction must be achieved as much as possible. -
Strong internal fixation: Provide sufficient stability to meet biomechanical needs through a precisely designed internal fixation system. -
Non-invasive surgical techniques: During surgery, strive to protect the blood supply to the fracture site and surrounding soft tissues, minimizing additional damage caused by the procedure. -
Early mobilization: With stable internal fixation support, patients can start muscle and joint activities as soon as possible to prevent complications caused by prolonged bed rest.
The AO perspective emphasizes that mechanical stability is the key to achieving fracture healing, and the precision of the operation and the quality of internal fixation directly affect the success of the treatment.
-
Protect soft tissue and blood supply: Minimize interference with the fracture area and surrounding soft tissue during surgery, and maintain blood supply. -
Appropriate internal fixation: Use materials with good biocompatibility and low elastic modulus to reduce stress shielding on bones and promote fracture healing. -
Minimally Invasive Surgery Technology: Using minimally invasive techniques to reduce the impact of surgery on the patient's overall condition and accelerate recovery.
Minimally Invasive Percutaneous Osteosynthesis (MIPO): In recent years, the development of minimally invasive surgical techniques aims to reduce damage to soft tissues during surgery, thereby preserving blood supply and promoting faster healing.
-
Protect local soft tissues: Reduce the fracture away from the site to preserve the attachment of local soft tissues. -
Do not insist on anatomical reduction of fracture fragments in comminuted fractures: unless it is an intra-articular fracture, there is no need to pursue perfect anatomical reduction. -
Use biocompatible materials: such as low elastic modulus materials, to reduce contact between internal fixation devices and bones, thereby minimizing stress shielding effects. -
Minimize surgical exposure time: Strive to shorten the duration of the surgery to reduce the overall impact on the patient.
Orthopedic external fixator frame and application
A骨外固定器 is actually a third type of fixation method between internal fixation and external fixation in orthopedics, which partially immobilizes fractures or dislocations with minimal trauma. It combines the advantages of both internal and external fixation. Compared to internal fixation, it causes less damage and has a lower wound infection rate. Compared to external fixation methods such as splints and plaster casts, it provides more reliable and stable fixation. However, it also has its own shortcomings and drawbacks. Therefore, when choosing to use a bone external fixator, one should strictly adhere to its indications and contraindications.
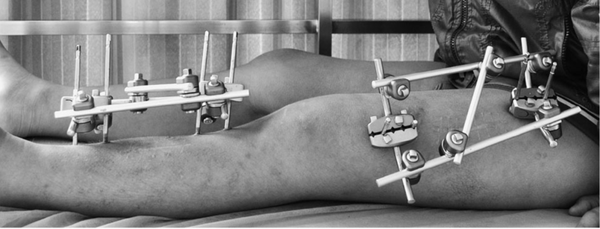
Unilateral external fixator
-
The fixation device is located on one side of the fracture and fixes the bone fragments through connecting rods and pins.
-
It is applicable for simpler fractures or when local skin conditions do not allow the use of circular or bilateral fixators.
Bilateral or multi-side external fixator
-
Definition: The fixation device is positioned on two or multiple sides of the fracture, providing more uniform support and stability through multiple connecting rods and pins.
-
Application: Suitable for complex fractures or reconstruction surgeries that require more stable fixation.
【Copyright and Disclaimer】The above information is collected and organized by PlastMatch. The copyright belongs to the original author. This article is reprinted for the purpose of providing more information, and it does not imply that PlastMatch endorses the views expressed in the article or guarantees its accuracy. If there are any errors in the source attribution or if your legitimate rights have been infringed, please contact us, and we will promptly correct or remove the content. If other media, websites, or individuals use the aforementioned content, they must clearly indicate the original source and origin of the work and assume legal responsibility on their own.
Most Popular
-
Amcor Opens Advanced Coating Facility for Healthcare Packaging in Malaysia
-
ExxonMobil and Malpack Develop High-Performance Stretch Film with Signature Polymers
-
Plastic Pipe Maker Joins Lawsuit Challenging Trump Tariffs
-
Pont, Blue Ocean Closures make biobased closures work
-
Over 300 Employees Laid Off! Is Meina Unable to Cope?